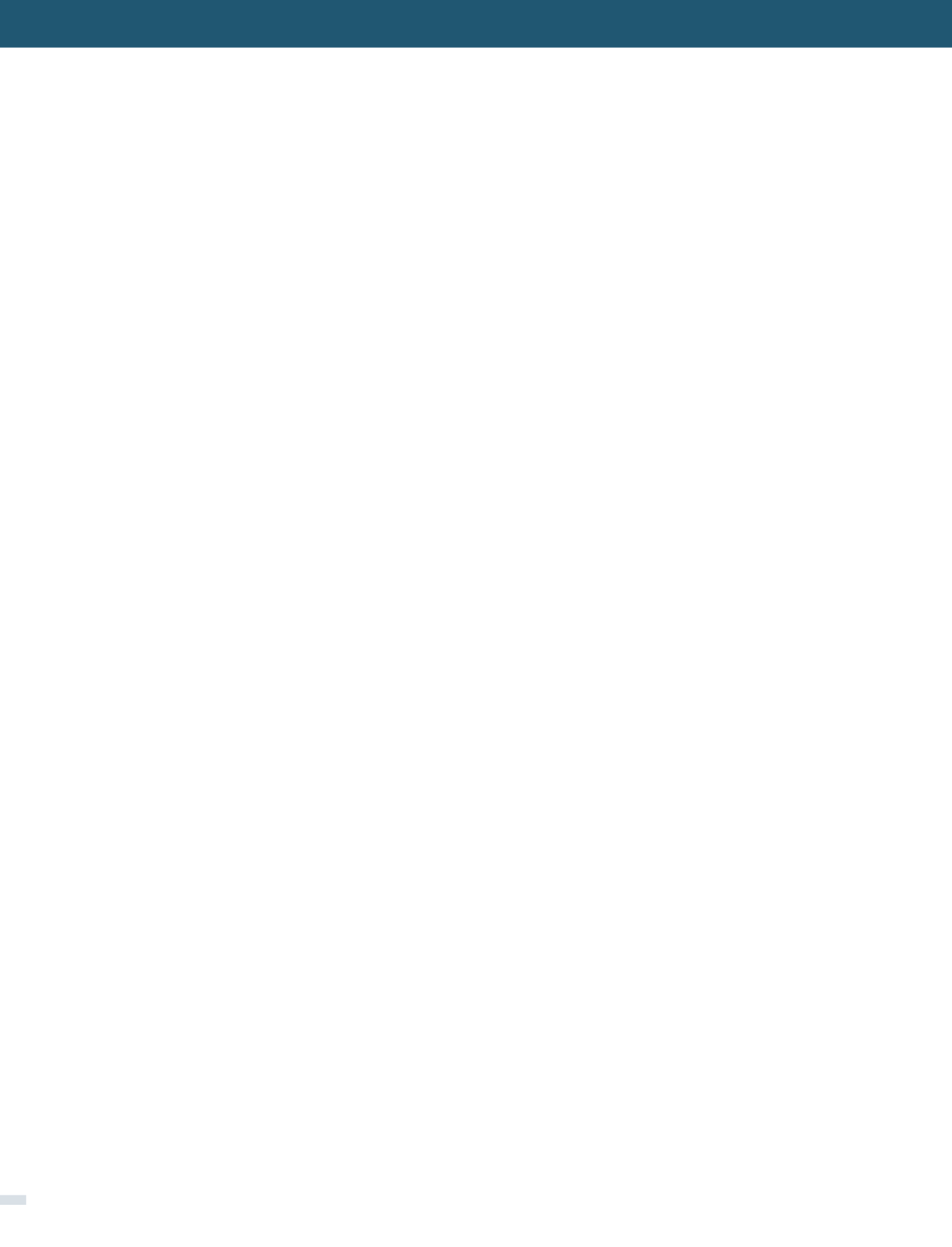
622
the organism may have a MIC of 32mg/L and potentially be
treated with a higher than standard dose of the antibiotic, or
the MIC may be much higher (e.g., 256mg/L), in which case
it would not be possible to obtain the required bactericidal
exposure without significantly increasing the risk of toxicity
to the patient. MIC data are most useful when considering
antibiotic pharmacodynamics because drug exposure is
always referenced to the MIC when deciding how much and
over what dosing interval to administer an antibiotic.
MIC testing can be conducted using various methods:
broth microdilution, macrodilution, agar dilution, Etest®
(BioMérieux, Durham, NC, USA), a type of diffusion test
using gradient technology, and finally with some automated
systems. The BD Phoenix™ Automated Microbiology System
(BD Diagnostics, Sparks, MD, USA) and Vitek® 2 (BioMérieux,
Durham, NC, USA) will also provide MIC results for an
antibiotic/bacteria combination, but only over a few dilution
ranges. For example, cefepime MICs for gram-negatives on
the BD Phoenix™ system test from 0.5 to 16mg/L, which
again would not inform the provider if an organism is
potentially treatment with a higher dose/prolonged infusion
at 32mg/L. When feasible, the use of broth microdilution or
Etest is preferred to collect data on MIC distributions locally
(by hospital or by unit), and can also be used for individual
patients with MDR infections to help optimize antibiotic
therapy, as both of these methodologies will provide for a
larger MIC range to be tested.
IMPLEMENTING OPTIMIZED REGIMENS BY THE ASP
ASPs can take two different approaches to optimizing the use
of an antibiotic. The traditional approach is to focus on the
antibiotic itself; each time it is prescribed, that antibiotic
is being optimized for that individual patient. The second
is to approach the treatment of the infection itself using
the most optimal strategy. With respect to implementing
an optimized antibiotic dosing regimen in the institution,
the latter strategy holds more merit. Before determining
which antibiotic and dosing regimen to apply optimization
to, it is critical to understand what the most likely causative
pathogens are for the infection (e.g., ventilator associated
pneumonia
) and the MICs for these most causative bacteria.
The ventilator associated
pneumonia
clinical pathway at
our hospital was instituted after collection of 8 months
of bacteria surveillance data and MIC testing (39).
Pharmacodynamic models were employed based on the
most frequent causative pathogen for which MIC data were
available,
P. aeruginosa
, to determine the choice of antibiotic
and dosage regimen that would provide the greatest
likelihood of obtaining its bactericidal pharmacodynamic
exposure. Both continuous and prolonged infusion regimens
as well as standard dosages were evaluated against the
P. aeruginosa
population. Due to increasing resistance in
certain ICUs at our hospital, high-dose prolonged infusion
regimens of cefepime or meropenem (2g every 8 hours
as 3 hour infusions) were required to achieve optimal
exposure, as these regimens would obtain a high likelihood
of attaining pharmacodynamic exposure against isolates
with MICs up to 32 and 16mg/L, respectively. In addition,
tobramycin 7mg/kg once daily was advocated due to
the frequency of multi-drug resistant organisms and the
MIC90 for the
P. aeruginosa
population remaining at 2mg/L.
Fluoroquinolones were strongly discouraged and reserved for
patients unable to get aminoglycosides. Finally, a high dose
vancomycin protocol was initiated aiming for trough values
in the range of 15-20 μg/ml to cover for MRSA. After the
protocol was initiated, we learned that our MRSA population
predominantly had vancomycin MICs of 1.5 to 2mg/L. As a
result, we now allow the prescriber to change therapy to
linezolid if a patient with MRSA is not improving by day 3 of
high-dose vancomycin therapy. These dosing regimens were
protocolized in the ICUs using a computerized provider order
set. Education was conducted for all providers, nurses, and
pharmacists on the background/justification of the program
and when to use it.
After 12 months of use, data were collected to evaluate the
impact (both clinical outcomes as well as compliance) of
the clinical pathway. Compliance was nearly 100%, and 94
patients were treated for ventilator associated
pneumonia
during that time. Compared with the 74 patients used as
historical controls, patients treated by the clinical pathway
with cefepime or meropenem optimized dosing regimens had
lower infection-related mortality (8.5% vs 21.6%, p=0.029),
were more likely to receive an antibiotic with activity against
the causative pathogen empirically (71.6%, vs 48.6%, p=0.007),
had less MDR superinfections (9.6% vs 27.0%, p=0.006) and
less infection related length of hospital stay (10.5 vs 23 days,
p
<
0.001). An economic analysis observed approximately
$40,000 (US$) savings per patient treated on the clinical
pathway (40). This program is still a mandatory protocol in
our ICUs, although we continue to make adjustments to
our antibiotics and dosing regimens after screening MICs
every couple of years. More recently, a prolonged infusion
piperacillin/tazobactam regimen (4.5g q6h as a 3 hour
infusion) has been implemented across our health system
based on MIC data, contemporary pharmacokinetics, and the
use of smart pumps across the system.
For the above clinical pathway, implementation was solely in
the ICUs, which made education and monitoring easier. We
also focused our optimization strategy around beta-lactams,
[REV. MED. CLIN. CONDES - 2016; 27(5) 615-624]